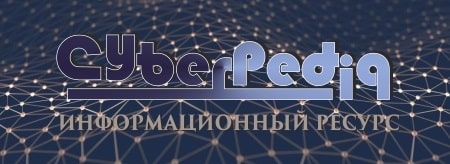
История создания датчика движения: Первый прибор для обнаружения движения был изобретен немецким физиком Генрихом Герцем...
Кормораздатчик мобильный электрифицированный: схема и процесс работы устройства...
Топ:
Эволюция кровеносной системы позвоночных животных: Биологическая эволюция – необратимый процесс исторического развития живой природы...
Оснащения врачебно-сестринской бригады.
Устройство и оснащение процедурного кабинета: Решающая роль в обеспечении правильного лечения пациентов отводится процедурной медсестре...
Интересное:
Инженерная защита территорий, зданий и сооружений от опасных геологических процессов: Изучение оползневых явлений, оценка устойчивости склонов и проектирование противооползневых сооружений — актуальнейшие задачи, стоящие перед отечественными...
Средства для ингаляционного наркоза: Наркоз наступает в результате вдыхания (ингаляции) средств, которое осуществляют или с помощью маски...
Берегоукрепление оползневых склонов: На прибрежных склонах основной причиной развития оползневых процессов является подмыв водами рек естественных склонов...
Дисциплины:
![]() |
![]() |
5.00
из
|
Заказать работу |
|
|
where:
§ m is the mass of the substance liberated at an electrode in grams
§ Q is the total electric charge passed through the substance
§ F = 96485 C mol−1 is the Faraday constant
§ M is the molar mass of the substance
§ z is the valency number of ions of the substance (electrons transferred per ion).
Note that M/z is the same as the equivalent weight of the substance altered.
For Faraday's first law, M, F, and z are constants, so that the larger the value of Q the larger m will be.
For Faraday's second law, Q, F, and z are constants, so that the larger the value of M/z (equivalent weight) the larger m will be.
In the simple case of constant-current electrolysis, leading to
and then to
where:
§ n is the amount of substance ("number of moles") liberated: n = m/M
§ t is the total time the constant current was applied.
In the more complicated case of a variable electrical current, the total charge Q is the electric current I () integrated over time
:
Here t is the total electrolysis time. Please note that tau is used as the current I is a function of tau.
In physics and chemistry, the Faraday constant (named after Michael Faraday) is the magnitude of electric charge per mole of electrons.[1] It has the currently accepted value 9.64853399(24) x 104 C mol-1
The constant F has a simple relation to two other physical constants:
where:
e ≈ 1.6021766×10−19 C;[2]
N A ≈ 6.022141×1023 mol−1.[3]
N A is the Avogadro constant (the ratio of the number of particles 'N' to the amount of substance 'n' - a unit mole), and e is the elementary charge or the magnitude of the charge of an electron. This relation is true because the amount of charge of a mole of electrons is equal to the amount of charge in one electron multiplied by the number of electrons in a mole.
The value of F was first determined by weighing the amount of silver deposited in an electrochemical reaction in which a measured current was passed for a measured time, and using Faraday's law of electrolysis. Research is continuing into more accurate ways of determining the interrelated constants F, N A, and e.
Other Common Units of Faraday's Constant
§ 96,485 J (96.485 kJ) per volt gram equivalent
§ 23.061 kcal per volt gram equivalent
§ 26.801 A·h/mol
Faraday unit of charge
Related to Faraday's constant is the "faraday", a unit of electrical charge. It is much less common than the coulomb, but sometimes used in electrochemistry.[5] One Faraday of charge is the magnitude of the charge of one mole of electrons, i.e. 96,485.3365(21) C.
Expressed in faradays, the Faraday constant F equals "1 faraday of charge per mole".
This faraday unit is not to be confused with the farad, an unrelated unit of capacitance (1 farad= 1 coulomb/1 volt).
Faraday's law of induction is a basic law of electromagnetism predicting how a magnetic field will interact with an electric circuit to produce an electromotive force (EMF). It is the fundamental operating principle of transformers, inductors, and many types of electrical motors, generators and solenoids.
|
The Maxwell–Faraday equation is a generalisation of Faraday's law, and forms one of Maxwell's equations.
§ Production of aluminium, lithium, sodium, potassium, magnesium, calcium
§ Coulometric techniques can be used to determine the amount of matter transformed during electrolysis by measuring the amount of electricity required to perform the electrolysis
§ Production of chlorine and sodium hydroxide
§ Production of sodium chlorate and potassium chlorate
§ Production of perfluorinated organic compounds such as trifluoroacetic acid
§ Production of electrolytic copper as a cathode, from refined copper of lower purity as an anode.
Electrolysis has many other uses:
§ Electrometallurgy is the process of reduction of metals from metallic compounds to obtain the pure form of metal using electrolysis. For example, sodium hydroxide in its molten form is separated by electrolysis into sodium and oxygen, both of which have important chemical uses. (Water is produced at the same time.)
§ Anodization is an electrolytic process that makes the surface of metals resistant to corrosion. For example, ships are saved from being corroded by oxygen in the water by this process. The process is also used to decorate surfaces.
§ A battery works by the reverse process to electrolysis.
§ Production of oxygen for spacecraft and nuclear submarines.
§ Electroplating is used in layering metals to fortify them. Electroplating is used in many industries for functional or decorative purposes, as in vehicle bodies and nickel coins.
§ Production of hydrogen for fuel, using a cheap source of electrical energy.
§ Electrolytic Etching of metal surfaces like tools or knives with a permanent mark or logo.
Electrolysis is also used in the cleaning and preservation of old artifacts. Because the process separates the non-metallic particles from the metallic ones, it is very useful for cleaning old coins and even larger objects.
Using a cell containing inert platinum electrodes, electrolysis of aqueous solutions of some salts leads to reduction of the cations (e.g., metal deposition with, e.g., zinc salts) and oxidation of the anions (e.g. evolution of bromine with bromides). However, with salts of some metals (e.g. sodium) hydrogen is evolved at the cathode, and for salts containing some anions (e.g. sulfate SO42−) oxygen is evolved at the anode. In both cases this is due to water being reduced to form hydrogen or oxidised to form oxygen. In principle the voltage required to electrolyze a salt solution can be derived from the standard electrode potential for the reactions at the anode and cathode. The standard electrode potentialis directly related to the Gibbs free energy, ΔG, for the reactions at each electrode and refers to an electrode with no current flowing. An extract from the table of standard electrode potentials is shown below.
In terms of electrolysis, this table should be interpreted as follows:
§ Oxidized species (often a cation) with a more negative cell potential are more difficult to reduce than oxidized species with a more positive cell potential. For example it is more difficult to reduce a sodium ion to a sodium metal than it is to reduce a zinc ion to a zinc metal.
§ Reduced species (often an anion) with a more positive cell potential are more difficult to oxidize than reduced species with a more negative cell potential. For example it is more difficult to oxidize sulfate anions than it is to oxidize bromide anions.
|
Using the Nernst equation the electrode potential can be calculated for a specific concentration of ions, temperature and the number of electrons involved. For pure water (pH 7):
§ the electrode potential for the reduction producing hydrogen is −0.41 V
· the electrode potential for the oxidation producing oxygen is +0.82 V.
Comparable figures calculated in a similar way, for 1M zinc bromide, ZnBr2, are −0.76 V for the reduction to Zn metal and +1.10 V for the oxidation producing bromine. The conclusion from these figures is that hydrogen should be produced at the cathode and oxygen at the anode from the electrolysis of water which is at variance with the experimental observation that zinc metal is deposited and bromine is produced.[6]The explanation is that these calculated potentials only indicate the thermodynamically preferred reaction. In practice many other factors have to be taken into account such as the kinetics of some of the reaction steps involved. These factors together mean that a higher potential is required for the reduction and oxidation of water than predicted, and these are termed overpotentials. Experimentally it is known thatoverpotentials depend on the design of the cell and the nature of the electrodes.
For the electrolysis of a neutral (pH 7) sodium chloride solution, the reduction of sodium ion is thermodynamically very difficult and water is reduced evolving hydrogen leaving hydroxide ions in solution. At the anode the oxidation of chlorine is observed rather than the oxidation of water since the overpotential for the oxidation of chloride to chlorine is lower than the overpotential for the oxidation of water to oxygen. Thehydroxide ions and dissolved chlorine gas react further to form hypochlorous acid. The aqueous solutions resulting from this process is calledelectrolyzed water and is used as a disinfectant and cleaning agent.
Electrolysis of water
One important use of electrolysis of water is to produce hydrogen.
2 H2O(l) → 2 H2(g) + O2(g); E0 = -1.229 V
Hydrogen can be used as a fuel for powering internal combustion engines by combustion or electric motors via hydrogen fuel cells (see Hydrogen vehicle). This has been suggested as one approach to shift economies of the world from the current state of almost complete dependence upon hydrocarbons for energy (See hydrogen economy.)
The energy efficiency of water electrolysis varies widely. The efficiency of an electrolyser is a measure of the enthalpy contained in the hydrogen (to under go combustion with oxygen, or some other later reaction), compared with the input electrical energy. Heat/enthalpy values for hydrogen are well published in science and engineering texts, as 144 MJ/kg. Note that fuel cells (not electrolysers) cannot utilise this full amount of heat/enthalpy, which has led to some confusion when calculating efficiency values for both types of technology. In the reaction, some energy is lost as heat, a useless byproduct. Some reports quote efficiencies between 50% and 70% for alkaline electrolysers; however, much higher practical efficiencies are available with the use of PEM and catalytic technology, such as 95% efficiency.[7][8] In the US there is still an occasional erroneous tendency to use the 'Lower Heating Value' for efficiencies. This value (becoming obsolete) does not represent the total amount of energy within the hydrogen, hence the efficiency appears lower than when using the more accurately defined values. The theoretical maximum considers the total amount of energy required for the formation of the hydrogen and oxygen from water. Note that (in more broader contexts of energy efficiency), these values refer only to the efficiency of converting electrical energy into hydrogen's chemical energy; the energy lost in generating the electricity is not included.
Single displacement reaction
|
From Wikipedia, the free encyclopedia
A single-displacement reaction, also called single-replacement reaction, is a type of oxidation-reduction chemical reaction when an element or ion moves out of one compound and into another - that is, one element is replaced by another in a compound. This is represented by the general reaction scheme:
A + BC → AC + B
This will most often occur if A is more reactive than B.
A and B must be either:
Different metals (hydrogen's behavior as a cation renders it as a metal here), in which case C represents an anion; or
Halogens, in which case C represents a cation.
In either case, when AC and BC are aqueous compounds (which is usually the case), C is a spectator ion.
Copper displaces silver in solution when a copper wire is dipped in a silver nitrate solution, and solid silver precipitates out.
In the activity or reactivity series, the metals with the highest propensity to donate their electrons to react are listed first, and the most unreactive metals are listed last. Therefore a metal higher on the list is able to displace anything on the list below it.
The order of activity for metals, from most reactive to least reactive, is
K, Li, Ba, Ca, Na, Mg, Al, Mn, Zn, Cr, Fe, Co, Ni, Sn, Pb, H2, Cu, Ag, Hg, Pt, Au.
Similarly, the halogens with the highest propensity to acquire electrons are the most reactive. The activity series for halogens, from highest to lowest, is F, Cl, Br,
Due to the free state nature of A and B, all single displacement reactions are also oxidation-reduction reactions, where the key event is the movement of electrons from one reactant to another. When A and B are metals, A is always oxidized and B is always reduced. Since halogens prefer to gain electrons, A is reduced (from a 0 to −1) and B is oxidized (from −1 to 0) when A and B represent those elements.
A and B may have different charge as ions and therefore some balancing of the equation may be necessary. For example the reaction between silver nitrate, AgNO3 (which contains an Ag+ ion), and zinc, Zn, forms silver, Ag, and zinc nitrate, Zn(NO3)2 (which contains a Zn 2+ ion).
2AgNO3(aq) + Zn(s) → 2Ag(s) + Zn(NO3)2(aq)
All simple metal with acid reactions are single displacement reactions. For example the reaction between magnesium, Mg, and hydrochloric acid, HCl, forms magnesium chloride, MgCl2, and hydrogen, H2.
Mg(s) + 2 HCl(aq) → MgCl2(aq) + H2(g)
Standard electrode potentials (reduction potentials
Each half-reaction has a standard electrode potential (E0cell), which is equal to the potential difference (or voltage) (E0cell) at equilibrium under standard conditions of an electrochemical cell in which the cathode reaction is the half-reaction considered, and the anode is a standard hydrogen electrode where hydrogen is oxidized: ½ H2 → H+ + e-.
The electrode potential of each half-reaction is also known as its reduction potential E0red, or potential when the half-reaction takes place at a cathode. The reduction potential is a measure of the tendency of the oxidizing agent to be reduced. Its value is zero for H+ + e− → ½ H2 by definition, positive for oxidizing agents stronger than H+ (e.g., +2.866 V for F2) and negative for oxidizing agents which are weaker than H+ (e.g. –0.763 V for Zn 2+).
For a redox reaction which takes place in a cell, the potential difference E0cell = E0cathode – E0anode
Historically, however, the potential of the reaction at the anode was sometimes expressed as an oxidation potential, E0ox = – E0. The oxidation potential is a measure of the tendency of the reducing agent to be oxidized, but does not represent the physical potential at an electrode. With this notation, the cell voltage equation is written with a plus sign E0cell = E0cathode + E0ox (anode)
|
|
|
Адаптации растений и животных к жизни в горах: Большое значение для жизни организмов в горах имеют степень расчленения, крутизна и экспозиционные различия склонов...
Типы сооружений для обработки осадков: Септиками называются сооружения, в которых одновременно происходят осветление сточной жидкости...
Таксономические единицы (категории) растений: Каждая система классификации состоит из определённых соподчиненных друг другу...
Автоматическое растормаживание колес: Тормозные устройства колес предназначены для уменьшения длины пробега и улучшения маневрирования ВС при...
© cyberpedia.su 2017-2024 - Не является автором материалов. Исключительное право сохранено за автором текста.
Если вы не хотите, чтобы данный материал был у нас на сайте, перейдите по ссылке: Нарушение авторских прав. Мы поможем в написании вашей работы!